Research Topic
When highly confined in nanophotonic structures, photons can interact very efficiently with artificial atoms. Such a setting is an ideal platform to observe and study giant non-linearities of light, i.e. effects that differs depending on the photon number, and can be used to perform quantum processing of information.
Quantum nanophotonics
Quantum nanophotonics blends two disciplines: expertise in solid-state physics and optics. Such combination allows for example to achieve highly coherent quantum dots, assimilated to simple quasi-perfect two-level quantum systems, highly coupled to light through high-quality nanophotonic structures, such as cavities, photonic crystals, or even simple waveguides.
This efficient interaction between light and matter enables one to reach coupling probabilities close to unity between each single photon and a quantum emitter. This results in very efficient and coherent light-matter interactions that allow us to observe phenomena such as the quasi-deterministic generation of single photons, spin-photon entanglement, as well as giant non-linearities. The latter are called this way as they exhibit sensitivities at the single photon level. An example may be the single-photon saturation of a two-level emitter.
Indeed, when photons are sent to a two-level quantum scatterer, interference occurs between the input and the scattered field from the emitter, causing the single photons to get reflected. By varying the laser frequency, one can then observe an extinction of the transmission at the resonance. The higher the light-matter coupling is, and the more ideal the single emitter is, the more single photon gets reflected.
A transmission dip of 10%, sensitive to the single photon level had thus been demonstrated for the first time by the Lodahl group in 2015, where I was working as a postdoc. By working on new structures, and by stabilizing the charge environment around the quantum dot, we have been able to get closer to near-perfect “two-level atoms” and have been able to observe transmission extinctions of 50% in waveguides and 80% in photonic crystals, still saturable at the single photon level. These structures can already be used as quantum photon switches.
These nonlinearities can also be used to create photon sorters: single photons are reflected, while photon pairs are transmitted, and experimental schemes to create CZ quantum gates or Bell state analyzers have already been proposed. For all this, it is necessary to understand and analyze the behavior of multiphoton quantum states, which are much more complex. We worked on modelling the action of a two-level emitter on two-photon states and implemented an experiment to detect such response.
Associated Publications
Coherent quantum optics, where the phase of a photon is not scrambled as
it interacts with an emitter, lies at the heart of many quantum optical
effects and emerging technologies. Solid-state emitters coupled to
nanophotonic waveguides are a promising platform for quantum devices, as
this element can be integrated into complex photonic chips. Yet,
preserving the full coherence properties of the coupled
emitter-waveguide system is challenging because of the complex and
dynamic electromagnetic landscape found in the solid state. Here, we
review progress toward coherent light-matter interactions with
solid-state quantum emitters coupled to nanophotonic waveguides. We
first lay down the theoretical foundation for coherent and nonlinear
light-matter interactions of a two-level system in a
quasi-one-dimensional system, and then benchmark experimental
realizations. We discuss higher order nonlinearities that arise as a
result of the addition of photons of different frequencies, more complex
energy level schemes of the emitters, and the coupling of multiple
emitters via a shared photonic mode. Throughout, we highlight protocols
for applications and novel effects that are based on these coherent
interactions, the steps taken toward their realization, and the
challenges that remain to be overcome.
Single photons role in the development of quantum science and
technology. They can carry quantum information over extended distances
to act as the backbone of a future quantum Internet(1) and can be
manipulated in advanced photonic circuits, enabling scalable photonic
quantum computing(2,3). However, more sophisticated devices and
protocols need access to multi-photon states with particular forms of
entanglement. Efficient light-matter interfaces offer a route to
reliably generating these entangled resource states(4,5). Here we
utilize the efficient and coherent coupling of a single quantum emitter
to a nanophotonic waveguide to realize a quantum nonlinear interaction
between single-photon wavepackets. We demonstrate the control of a
photon using a second photon mediated by the quantum emitter. The
dynamical response of the two-photon interaction is experimentally
unravelled and reveals quantum correlations controlled by the pulse
duration. Further development of this platform work, which constitutes a
new research frontier in quantum optics(6), will enable the tailoring of
complex photonic quantum resource states.
Coherent photon-emitter interfaces offer a way to mediate efficient
nonlinear photon-photon interactions, much needed for quantum
information processing. Here we experimentally study the case of a
two-level emitter, a quantum dot, coupled to a single optical mode in a
nanophotonic waveguide. We carry out few-photon transport experiments
and record the statistics of the light to reconstruct the scattering
matrix elements of one- and two-photon components. This provides direct
insight to the complex nonlinear photon interaction that contains rich
many-body physics.
Establishing a highly efficient photon-emitter interface where the
intrinsic linewidth broadening is limited solely by spontaneous emission
is a key step in quantum optics. It opens a pathway to coherent light
matter interaction for, e.g., the generation of highly indistinguishable
photons, few photon optical nonlinearities, and photon-emitter quantum
gates. However, residual broadening mechanisms are ubiquitous and need
to be combated. For solid-state emitters charge and nuclear spin noise
are of importance, and the influence of photonic nanostructures on the
broadening has not been clarified. We present near-lifetime-limited
linewidths for quantum dots embedded in nanophotonic waveguides through
a resonant transmission experiment. It is found that the scattering of
single photons from the quantum dot can be obtained with an extinction
of 66 +/- 4%, which is limited by the coupling of the quantum dot to
the nanostructure rather than the linewidth broadening. This is obtained
by embedding the quantum dot in an electrically contacted nanophotonic
membrane. A clear pathway to obtaining even larger single-photon
extinction is laid out; i.e., the approach enables a fully deterministic
and coherent photon-emitter interface in the solid state that is
operated at optical frequencies.
On-chip chiral quantum light-matter interfaces, which support
directional interactions, provide a promising platform for efficient
spin-photon coupling, nonreciprocal photonic elements, and quantum logic
architectures. We present full-wave three-dimensional calculations to
quantify the performance of conventional and topological photonic
crystal waveguides as chiral emitter-photon interfaces. Specifically,
the ability of these structures to support and enhance directional
interactions while suppressing subsequent backscattering losses is
quantified. Broken symmetry waveguides, such as the nontopological
glide-plane waveguide and topological bearded interface waveguide are
found to act as efficient chiral interfaces, with the topological
waveguide modes allowing for operation at significantly higher Purcell
enhancement factors. Finally, although all structures suffer from
backscattering losses due to fabrication imperfections, these are found
to be smaller at high enhancement factors for the topological waveguide.
These reduced losses occur because the optical mode is pushed away from
the air-dielectric interfaces where scattering occurs, and not because
of any topological protection. These results are important to the
understanding of light-matter interactions in topological photonic
crystal and design of efficient, on-chip chiral quantum devices.
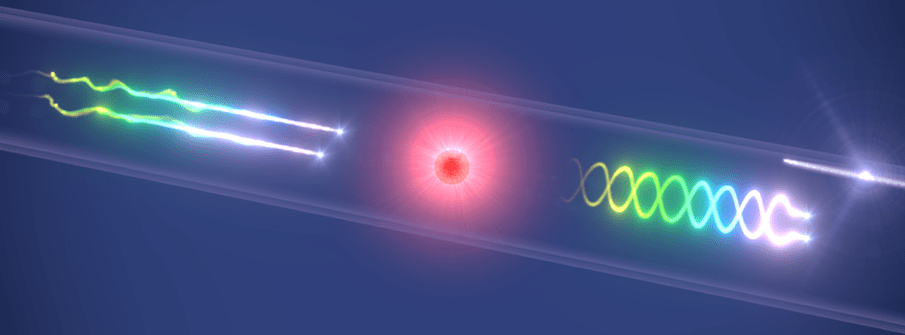